The element that can make bones glow
- Published
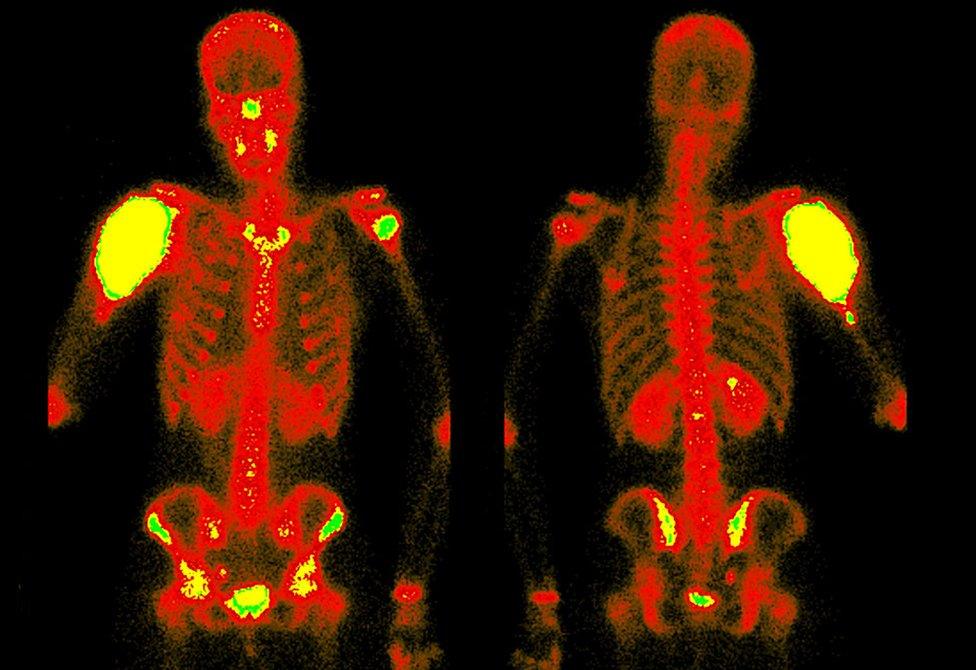
Photo: Science Photo Library
Scientists spent years searching for Technetium, a chemical element that came, in time, to play a crucial role in medical diagnosis. So crucial in fact that a new search is already under way - to find a fresh and reliable source of the elusive metal substance, before current sources dry up.
"It is an element beloved of chemists," explains chemist Andrea Sella of University College London, "because almost none of us has seen it".
The tale of its discovery is something of a shaggy dog story, beginning with the father of the periodic table, the 19th Century Russian polymath Dmitri Mendeleev.
"He was the man who first came up with this systematic scheme for the elements," explains Sella. "He spotted four gaps in this table, and one of them was right in the middle, among the transition metals."
That gap - element number 43 - was for technetium. But nobody knew that at the time.
"The fact that there was this hole led to something like 70 years of desperate searching across the globe. This was the time when everyone was hoping to find fame and fortune by discovering an element, and no-one could find it."
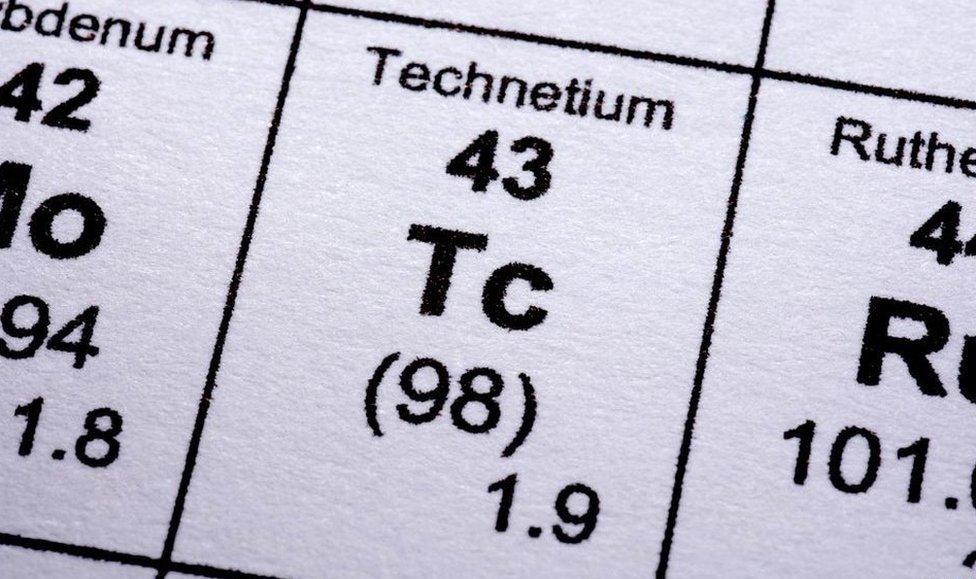
Photo: Thinkstock
Among the various candidates put forward were "davyum", derived from a platinum-bearing ore in the Ural mountains and named, in 1877, after the famous British chemist Humphry Davy.
Another was "lucium", a substance found in 1896 in a reddish-brown mineral called monazite, which turned out to be an impure sample of a rare earth element, yttrium.
Yet another was "nipponium", extracted by a Japanese researcher in 1908 from the rare mineral, thorianite. This later turned out to be element number 75, rhenium - another of the gaps in Mendeleev's table.
In 1925, came the most controversial claimant. The German husband-and-wife team Walter and Ida Noddack claimed to have glimpsed element 43 as part of a nuclear reaction, and named it "masurium" after the Masurian lakes, where Walter was born.
But no other scientists were able to reproduce their experiment, and science historians continue to debate to this day whether they could have spotted it or not.
In any case, the naming rights fell instead to an Italian duo, Carlo Perrier and Emilio Segre. Like the Noddacks, they sought the element among the weird zoo of matter created in nuclear reactions.
In 1936 they found it in a sample of another metal, molybdenum, which had been bombarded with particles by Ernest Lawrence using his cyclotron in California.
Because it was cooked up in a nuclear reaction, they named it after the Greek word "technos", meaning synthetic, or artificial.
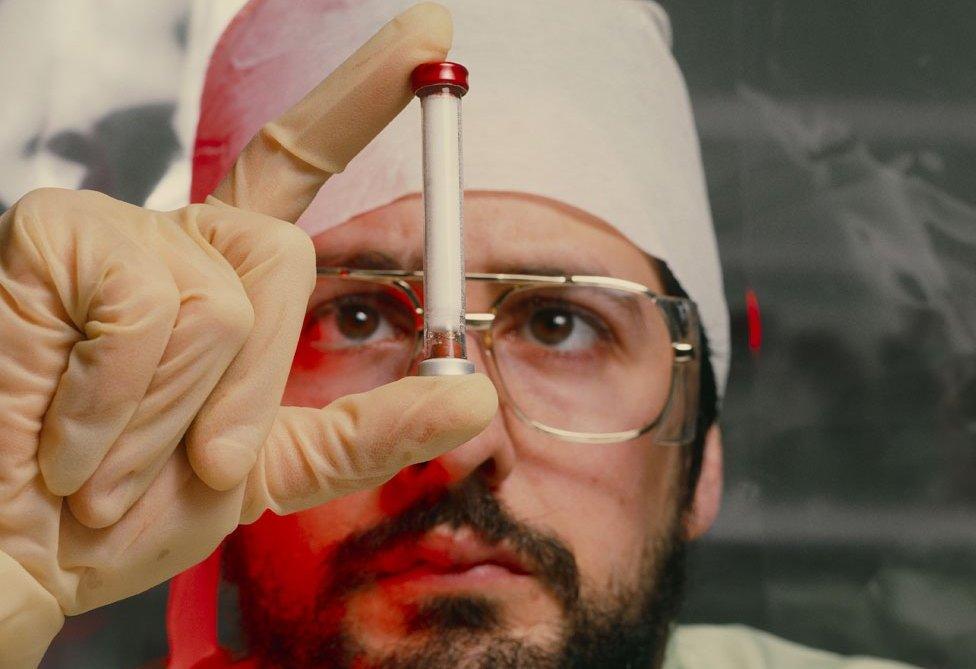
A sample of technetium. Photo: Science Photo Library
"When it was discovered, it was imagined that it would only ever be made artificially, and would not be found in nature," says Sella.
To understand why, it is useful to recall what an element is. The nucleus of an atom contains two types of particle - protons and neutrons.
The positively-charged protons determine the chemical properties of the atom - how it bonds with other atoms - and therefore what element it is.
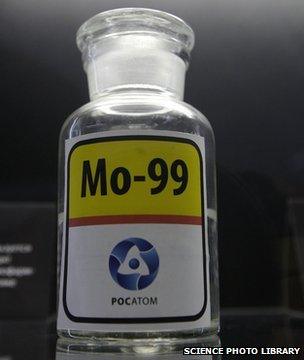
Sample of molybdenum
The neutrons have no charge, and do not affect the atom's chemistry. But that means two atoms of the same element can have different numbers of neutrons in them. Chemically, they are identical - there is just a difference in weight. These variants of the same element are called "isotopes".
But there is a limit to the number of isotopes. If the nucleus contains too few neutrons, or too many, it becomes unstable and breaks down radioactively, spitting out particles and rays, and often morphing into another element. Most elements only have one or a small handful of stable isotopes.
And that's where technetium comes in. Because it turns out that due to a quirk in the mathematics of atomic nuclei, every single isotope of technetium is unstable - all versions of technetium are radioactive and eventually decay into other elements.
That explains the 70-year wild goose chase.
It so happens, though, that some technetium does exist in the natural world.
In a bizarre twist, in 1972 it was discovered in Gabon that a natural nuclear reaction had taken place in a uranium deposit almost two billion years ago, and there were still tiny traces remaining of the radioactive elements created in that reaction, including technetium.
One special isotope of technetium has a very useful application.
That isotope is technetium-99m. The 99 refers to the 43 protons plus 56 neutrons in its nucleus. And the m refers to a specific configuration of those particles.
The result is an atom that decays within a few hours, generating gamma rays - radiation that can be used to produce medical images.
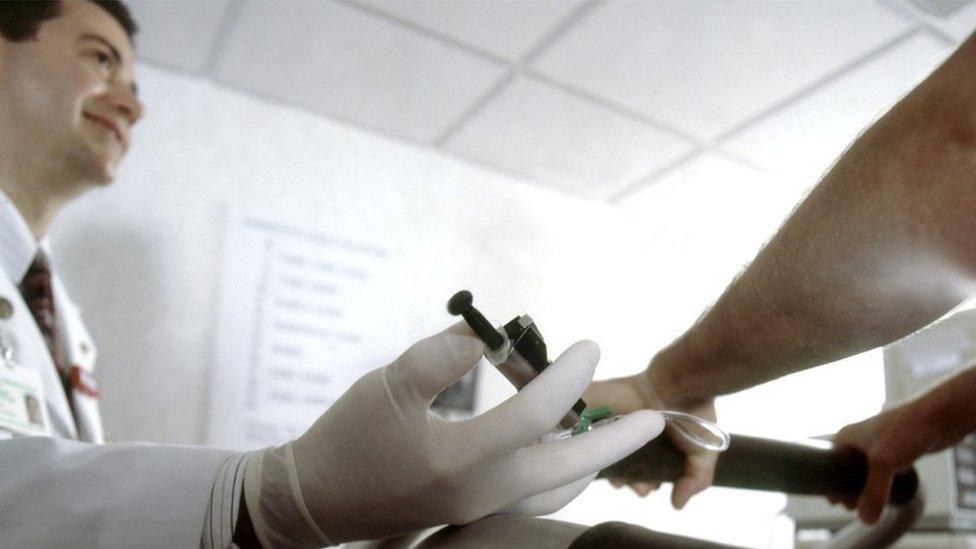
Technetium being injected into a patient: Science Photo Library
At UCL Hospital in London, Sarah is about to undergo just such a scan to look at her spine, ahead of an operation to fuse two of her vertebrae.
First, she has to be injected with the technetium. Her doctor assays the dose - the technetium is continually decaying, so it is important to check that it is still sufficiently radioactive to produce an image, but not enough to be harmful.
The injection does not just contain technetium. Each radioactive atom is attached to a "ligand" - a chemical that ensures the technetium reaches the right part of Sarah's body.
"If you were to give the radioactivity on its own, it would probably end up in the patient's thyroid and a little bit in the stomach, and also salivary glands," the doctor explains.
In this case, the ligand is a phosphonate - a type of salt that is absorbed from the blood by bones.
And this is the magic of the technetium scan. It generates a telling pattern of hot spots - hives of activity where the bone may be repairing itself and has therefore absorbed more phosphonate from the blood - and cold spots where the bone may be dead.
"If you were to X-ray two people - one is dead and one is alive, but they have no fractures - you wouldn't be able to tell which is dead," explains Sarah's doctor. "In nuclear medicine, if that part of the body doesn't work and there's no blood flow there, you will not see it."
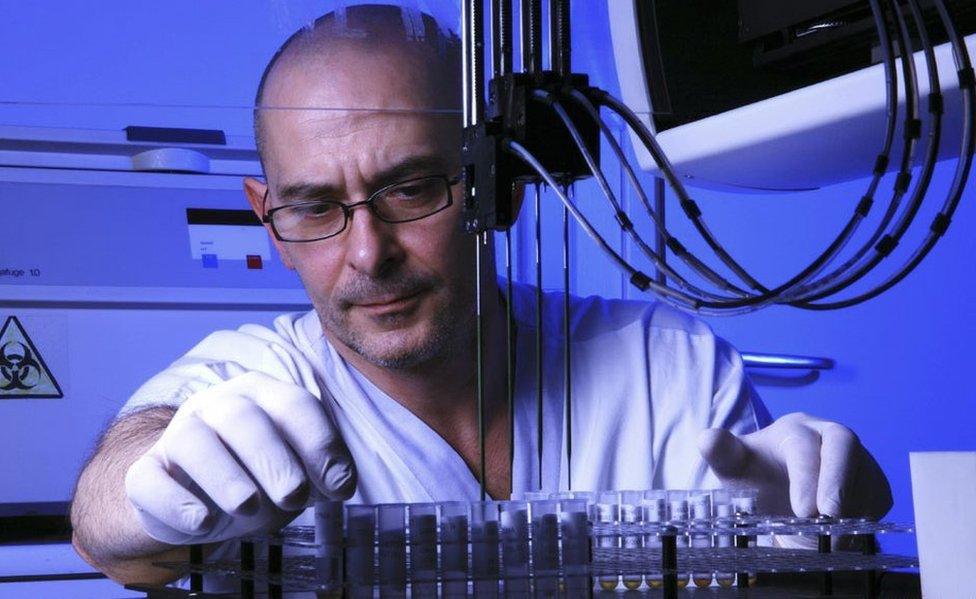
Photo: Science Photo Library
Before she can be scanned, Sarah has to wait four hours for the technetium to find its way to her spine, during which time she is advised to avoid close contact with small children as she is mildly radioactive.
After the four hours, there is a short window of time during which the scan can be done. Wait too long, and the technetium will decay away altogether - it has a half-life of six hours - or be flushed out of Sarah's body.
Upon her return, she is slowly put through a full-body scanner that detects the gamma-ray emissions, and uses that data to create a three-dimensional image of what is happening in her spine.
Bone is just one material that technetium can be used to peer at. By attaching it to other chemical ligands, this radioactive marker can be towed through the body's metabolic pathways to other organs - the spleen, lungs, liver, lymph glands, heart.
"If you find a decent target - a protein in the body you can follow - then you can monitor anything," explains Dr Kerstin Sander, a nuclear medicine researcher attached to the hospital.
Moreover, it's not just the ligand that can be switched. The technetium-99m itself is merely the most versatile and commonly used of an entire suite of radioactive isotopes, each of which has a different half-life, and different radioactive and chemical properties, attuned to detecting particular diseases in the body.

Common medical radioisotopes
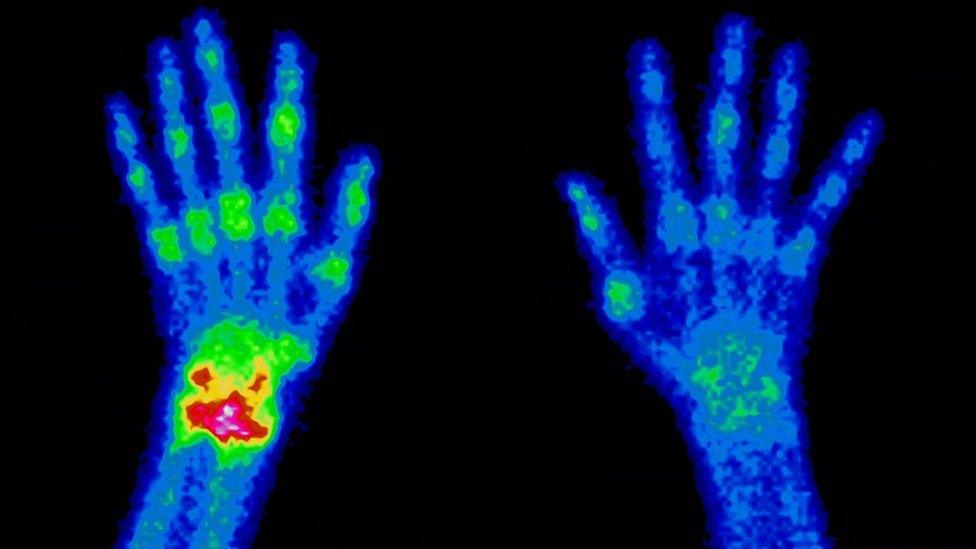
Photo: Science Photo Library
Technetium-99m - the most common radioisotope for diagnosis, accounting for over 80% of scans
Iodine-123 - diagnosis, particularly of the thyroid, and of neurological disease (such as Alzheimer's and Parkinson's)
Iodine-131 - treating thyroid disease, including cancer
Fluorine-18 - imaging malignant tumours
Thallium-201 - monitoring the heart during exercise
Gallium-67 - imaging tumours and locating inflammatory lesions
Indium-111 - locating blood clots, inflammation and rare cancers
Xenon-133 - inhaled for studying lung ventilation
Chromium-51 - monitoring blood cells, diagnosing gastrointestinal bleeding
Lutetium-177 - diagnosing and treating small cancer tumours
Yttrium-90 - treating cancer and relieving pain in arthritis
For a complete list, see the Radiochemistry Society, external

The tricky bit, on which Sander's research is focused, is working out how to attach the radioactive atom to the ligand.
But it's not just imaging that Sander hopes the chemicals she is developing can be used for. They might also in the future be used by pharmaceutical companies to research the effects of experimental medicines.
By attaching a radioisotope to a prototype drug, the researchers may be able to follow the path the drug takes through the body, helping them to decide at an early stage whether it has potential.
"Rather than spending 10-20 years optimising a new drug, you could explore some properties much, much earlier," she says.
And then there's radiotherapy. You can replace the technetium with something that emits far more powerful radiation that will destroy surrounding tissues, in particular cancer cells.
"It is like targeted chemotherapy, and it approaches more and more this dream of the 'magic bullet'. We can use antibodies, and then it becomes very targeted."
That's the great hope for the future. Back in the here-and-now, there is a different challenge to deal with - making sure we don't run out of the stuff.
To understand why, you need to follow the element back to its source. The technetium is produced by the decay of another radioactive isotope - molybdenum-99.
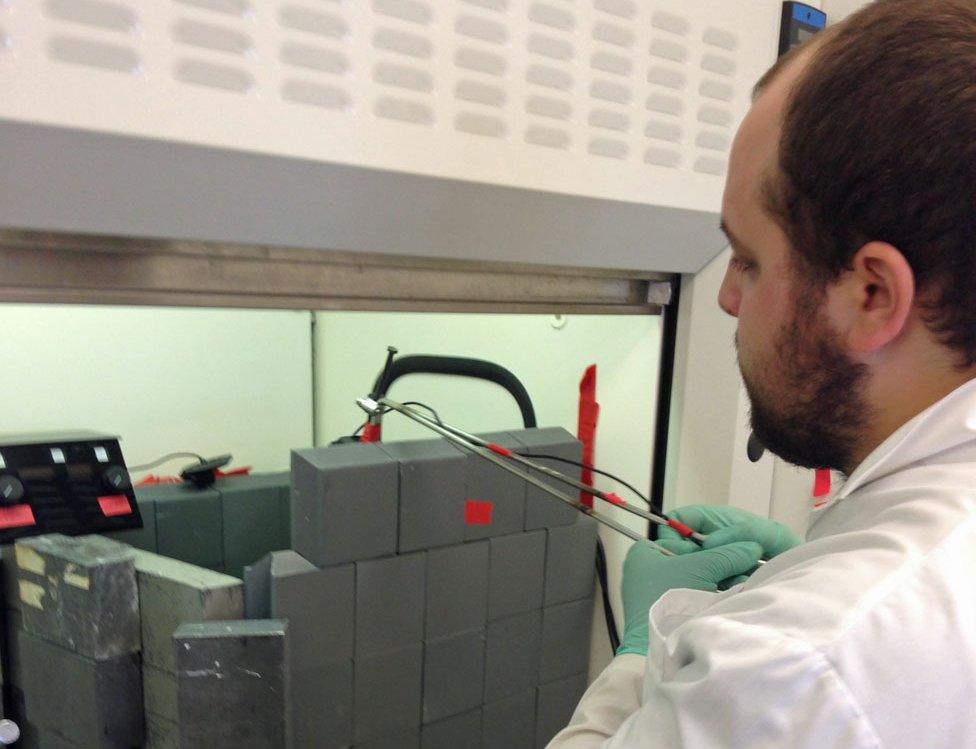
Milking the molybdenum
The UCL hospital keeps stocks of this parent isotope in canisters called "cows". The molybdenum can be regularly "milked" by passing salty water over it, washing out the day's yield of technetium.
Each successive milking produces less technetium, as the molybdenum itself decays away. And after a week the cow is exhausted, and is put in storage until it has lost sufficient radiation that it can safely be shipped back to the Netherlands, where UCL's cows are manufactured.
And the Netherlands is where all it begins. It is the site of one of two ageing nuclear facilities that provide the bulk of the world's molybdenum-99. The other is the Chalk River reactor in Canada.
Which brings us to the problem - occasionally, these reactors have to be shut down unexpectedly for maintenance. And technetium is not a commodity that can be stockpiled - it just decays away.
"What happens now if one of these reactors goes down, is that it creates a global shortage, and then doctors cannot get the images they need to diagnose disease," explains Dr Jeff Martin, physics professor at the University of Winnipeg. "And that's a huge problem."
The first such incident at Chalk River in 2007 caused such an acute crisis that the Canadian parliament passed an emergency law forcing the reactor to be reopened, and the chair of the Canadian Nuclear Safety Commission was fired.
But Chalk River is due to close permanently in 2018. There are a dozen other antiquated reactors dotted around the world that also produce medical isotopes. But it is unclear whether they can pick up the slack, particularly given the problems of shipping a radioactive material.
So Martin is engaged in one of several projects around the world that aims to provide an entirely new source of technetium.
"We're proposing a more green alternative, using a low-energy electron accelerator to take molybdenum 100 and knock neutrons out of it," he says.
Martin claims this has several advantages, besides the obvious one of alleviating the planet's uncomfortable reliance on the creaking Chalk River reactor.
It obviates the need to deal with enriched uranium - the fissile material for nuclear bombs - which is smashed up in the Chalk River reactor to produce molybdenum-99, as well as other far more dangerously radioactive materials.
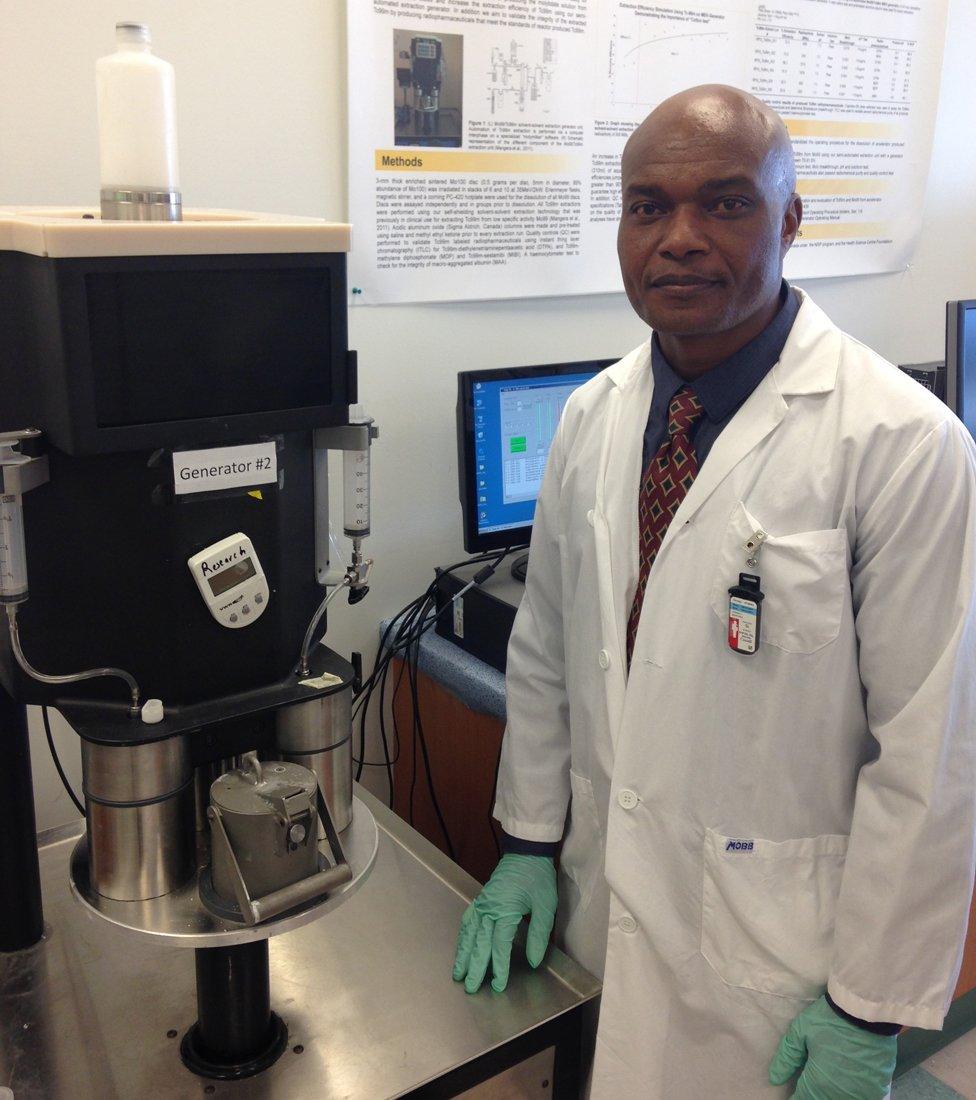
Dr Kennedy Mangera
"It is very easy to turn [the accelerator] off, and nothing is radioactive afterwards except the technetium," Martin claims.
These accelerators - smaller, safer, and more manageable than reactors - would also address another big problem.
"Speed is very important when we are handling technetium," explains Dr Kennedy Mangera, director of radiopharmacology at the Winnipeg Health Sciences Centre, and one of Martin's collaborators.
"It takes about 45 minutes to transport the product to the hospital. Traffic jams are problematic, because you are losing the product by the minute."
The parent isotope in the cows, the molybdenum-99, decays more slowly. It takes just under three days for half of it to disappear, rather than the mere six hours of technetium-99m.
But locating the accelerator a short drive from the hospital - as opposed, for example, to a half-day's journey across the English Channel from the Netherlands - would still avoid wasting a significant amount of material, as well as the need to move a radioactive material through ports, airports and across international borders.
In other words, an element that until a few decades ago scarcely existed on this planet may soon become available more-or-less on tap.

Technetium: key facts
First element to be artificially produced
Discovered in 1937 in a sample of molybdenum that had been bombarded by deuterons in the cyclotron at The University of California, Berkeley
Looks like platinum but is usually obtained as a grey powder
Source: Encyclopaedia Britannica

Subscribe to the BBC News Magazine's email newsletter to get articles sent to your inbox.