The element that causes arguments
- Published
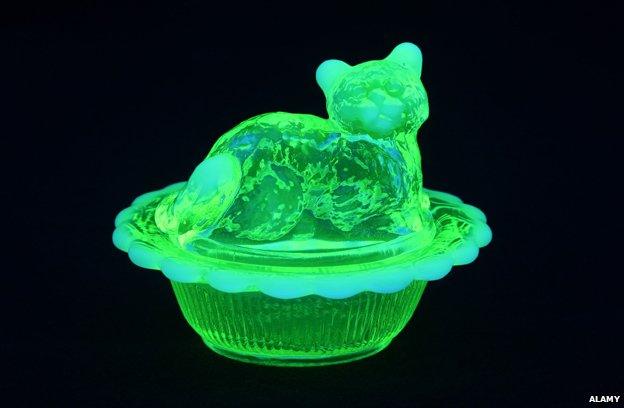
Uranium is the most divisive of elements.
When, in 1938, Otto Hahn first discovered the astonishing amounts of energy that could be released by splitting a single uranium atom, he opened the way not only to a potentially unlimited source of electricity, but also to the atomic bomb.
Today, the element's potential poses a new conundrum, one that has split environmentalists right down the middle.
Ironically, the first uses of uranium hardly even hinted at its incredible potential.
On a laboratory table in the chemistry department of University College London, Professor Andrea Sella has lined up a selection of greenish-yellow glassware - a salt cellar, a wineglass.
The zesty colour was, he tells me, particularly fashionable in the late Victorian period because of an extraordinary quality it possesses.
Sella shuts off the laboratory lights and switches on an ultraviolet bulb.
Suddenly the row of glasses lights up with an eerie fluorescence. The colour and the extraordinary glow is a result of the uranium salts in the glass, he explains.
This phenomenon both delighted and unsettled the Victorians. It was felt, even by some of the scientists investigating the properties of uranium, that the mysterious colours and lights hinted at some link with the world of the supernatural.
Only at the very end of the 19th Century was it discovered that that uranium did indeed have some otherworldly properties.
In 1896, Henri Becquerel found that rays emanating from uranium salts penetrated paper and caused fogging of an unexposed photographic plate. It was his doctoral student Marie Curie who named this property "radioactivity", taking the prefix "radio" from the Greek word meaning beam or ray.
The instability of the uranium atom is the source of this mysterious power. Uranium, with 92 protons, is the largest naturally occurring element on Earth, and its oversized nucleus is liable to break apart, shooting out "alpha" particles - bundles of two neutrons and two protons.
These particles are actually the nuclei of helium atoms, and it is because of the radioactive decay of uranium and other unstable elements that helium exists on Planet Earth.
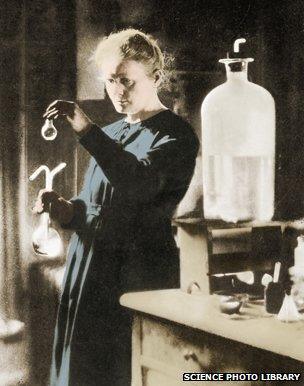
Alpha particles tear out of the uranium nucleus like shrapnel from an explosion. These tiny missiles travel at incredible speed - 10,000 miles (16,093km) a second. By the standards of radiation it isn't very dangerous, a sheet of paper is enough to protect the body from alpha radiation.
But each time an unstable element like uranium spits out a particle of radioactivity it "decays", transforming into another element. So uranium becomes thorium, which in turn becomes protactinium, until finally it decays into lead.
These decay elements produce other forms of radiation - beta and gamma - which can penetrate the human body, wreaking terrible damage as they go.
As they smash into cells they can kill them - that's what causes radiation sickness. Or they can disrupt our cells. More often than not our bodies repair themselves, but damaged cells sometimes start to proliferate wildly - that's what cancer is - or they can cause genetic mutations that we pass on to our children.
Marie Curie never fully appreciated the health risks of radiation - this is a woman who is said to have kept a glowing phial of radioactive isotopes beside her bed as a nightlight. Yet she, and many of her colleagues, would die of illnesses related to their exposure.
Radiation may be dangerous, but every time a radioactive atom fires out one of these little missiles there is a potentially very useful by-product (besides helium) - heat. And the heat produced by uranium is still playing a crucial role in shaping the physical environment of our world.
It is estimated that the slow decay of uranium and other radioactive elements is the source of around half the heat inside the Earth - the rest is left over from the formation of the planet.
That means uranium and its ilk have literally shaped the Earth as we know it. Their thermal legacy helps the power convection currents that are the source of the Earth's magnetic field, and also drives the movement of the tectonic plates that form the surface of the Earth. That tectonic movement has sculpted the landmasses upon which we all live.

Uranium: Key facts
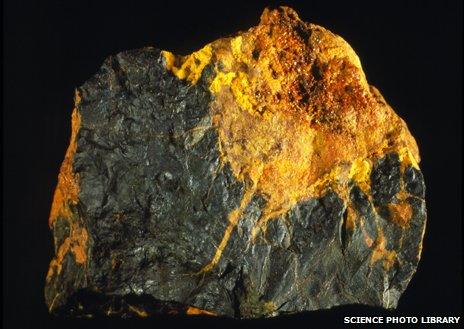
Uraninite (pitchblende) and gummite
Radioactive chemical element that is dense, hard and silvery white in colour
Chemical symbol for uranium is U
Constitutes about two parts per million of Earth's crust
First discovered in 1789 by German chemist Martin Heinrich Klaproth in a mineral called Pitchblende, and named after the planet Uranus

The ability of our species to unlock the power of uranium atoms derives from a related property of this insecure element.
In the 1930s scientists discovered that if you fire a neutron - an uncharged subatomic particle - into certain uranium atoms, you could split them into two, releasing a huge amount of energy along the way. This is called fission, from the Latin for "split".
The splitting of the atom represents a watershed in history, the first step in the harnessing of a hitherto unimaginable power.
Things moved very quickly after that first discovery. The world was on the verge of war and both the Americans and Germans realised it might be possible to use fission to create devastating new bombs.
That's because fission can be used to create a nuclear chain reaction. Every time a uranium atom is split it releases three neutrons which can go on to split further fissile nuclei, releasing yet more neutrons - with explosive consequences.
The challenge for the scientists racing to develop these terrifying new weapons was getting hold of enough fissile material.
Like other elements, uranium occurs in several slightly different forms known as "isotopes", which differ from each other in the number of neutrons in the nucleus. Natural uranium contains a mix of two main isotopes. By far the most common is uranium-238 which does not readily split. It accounts for 99.3% of the uranium found on earth. The remaining 0.7% is the fissile type, uranium-235.
In 1942 a team from the American Manhattan Project, led by the Italian physicist Enrico Fermi, built the world's first nuclear reactor on the floor of a squash court on the campus of the university of Chicago. It was known as "Chicago Pile-1" and Fermi used it to create the first self-sustaining chain reaction.
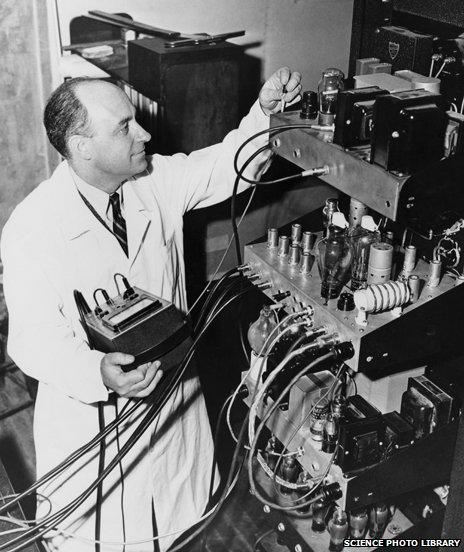
He showed that even natural uranium with its very low proportion of fissile material could be used to create a chain reaction. The trick was the use of graphite as a "moderator". Moderators make chain reactions more likely by slowing down the neutrons, which gives them a better chance of splitting other nuclei.
Bombs, though, are not the place for moderation. The uncontrolled nuclear reactions in atomic bombs require a high concentration of fissile material.
But separating uranium-235 from uranium-238 is very difficult - they are chemically nearly identical and have almost the same mass. It is possible using centrifuges, but centrifuge technology was only in its infancy. The Manhattan Project scientists worried they would not be able to produce enough of the stuff.
Fermi's nuclear reactor offered an alternative route to a bomb.
When a neutron hits one of the non-fissile uranium-238 nuclei, it can turn it into a new element, plutonium. Plutonium nuclei are fissile and the first nuclear reactors around the world became factories to convert uranium into plutonium for bomb programmes.
The success of the Manhattan Project was marked in truly horrific fashion when the Americans dropped atomic bombs - one made with uranium, the other with plutonium - on the Japanese cities of Hiroshima and Nagasaki in August 1945.
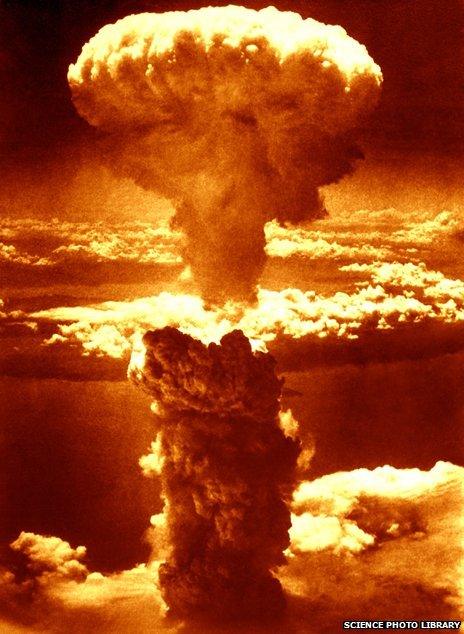
The bombs killed more than 150,000 people and within days the Japanese had surrendered, ending World War Two.
What followed was a great standoff. For decades the world was locked into Cold War. Conflict was contained because the consequences were now so great. This gave rise to the doctrine of "mutually assured destruction", and with it one of the most appropriate acronyms in history: MAD.
MAD had the lunatic effect of compelling both sides to develop ever more fearsome weapons to ensure the balance of power.
But, at the same time, attention turned to more peaceable uses for nuclear fission.
Generating power had been an afterthought with the early reactors. They needed to be cooled, and using the gas that cooled them to drive turbines was good public relations.
In the 1950s a new strand of nuclear research developed to look at the possibility of developing nuclear reactors specifically to generate electricity. Today, some 10% of the world's electricity is generated from the fission of uranium atoms.
Nuclear power stations are spookily quiet.
All you can hear, even deep inside the Sizewell B plant on the Suffolk coast, is a gentle hum.
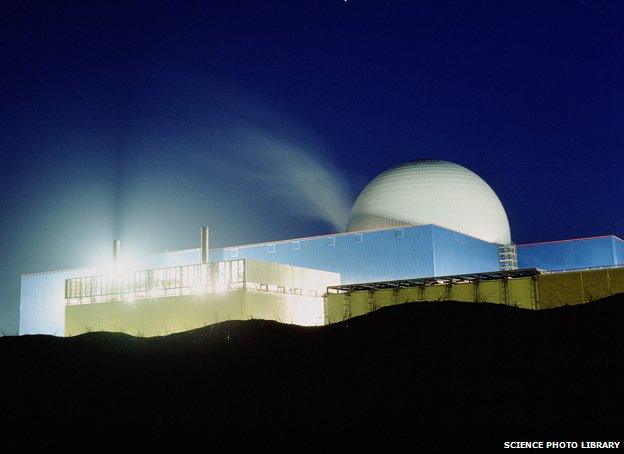
"Boring is good," says Colin Tucker who is in charge of safety at the plant.
But the hellish miracle at the heart of a modern reactor is far from boring. Inside the reactor core 1,000,000,000,000 (1 trillion) uranium atoms are splitting every second, he says.
Every day the controlled nuclear reaction at Sizewell B generates heat with the equivalent of more than three times the energy given off by the bomb that destroyed Hiroshima.
All that energy is contained within a couple of swimming pools' worth of super-heated water trapped under pressure in a steel cylinder.
And that's not the most hair-raising aspect of the process.
The station director, Jim Crawford, leads me through a seemingly endless series of aluminium lined corridors. We reach a particularly formidable security door where he tells me to clip on a personal Geiger counter.
I'm ushered into a giant concrete sarcophagus. A Hollywood set designer would struggle to contrive somewhere more eerie and ominous.
There is a fence overlooking a deep pool. Lights under the unusually blue water illuminate a silvery honeycomb. This is what is known as the spent fuel pond.
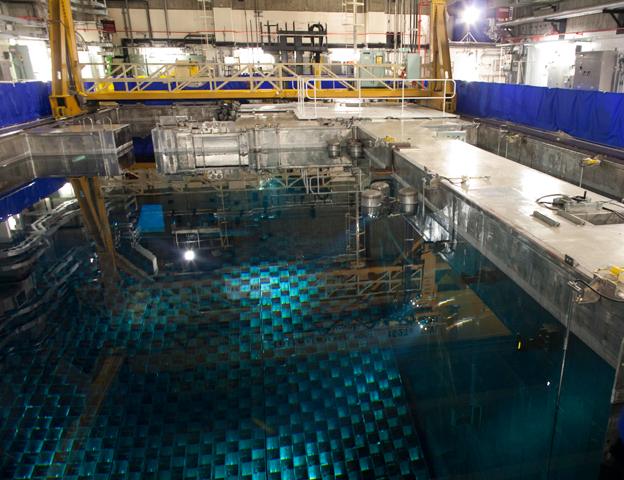
I stare down into the water.
"You are looking at some of the most radioactive material in the world," Crawford tells me.
The spent fuel pond is where the used uranium fuel rods are stored. Since these rods have been exposed to a nuclear reaction, many of the uranium-238 atoms have been transformed into even more radioactive plutonium.
I'm astonished how small it is, about 40m long and perhaps 15m wide. Sizewell B supplies between 3% and 4% of the UK's electricity, and has been in operation for almost two decades. Yet all the fuel used in all those years is stored in what is in effect a single Olympic swimming pool.
It is the danger posed by nuclear power and the waste it produces that has made the technology so unpopular around the world and explains why, for decades, environmentalists were implacably opposed to it.
But as evidence of the effects of global warming grows, the balance of risk is changing: the danger of nuclear disaster now needs to be weighed against the overwhelming consensus that greenhouse gas emissions are causing the climate to change.
Where once nuclear power united environmentalists in opposition, now the technology is beginning to split the green movement in two.
"Without nuclear the battle against global warming is as good as lost," claims Mark Lynas starkly. He is a former anti-nuclear campaigner who is now an ardent champion of the technology.
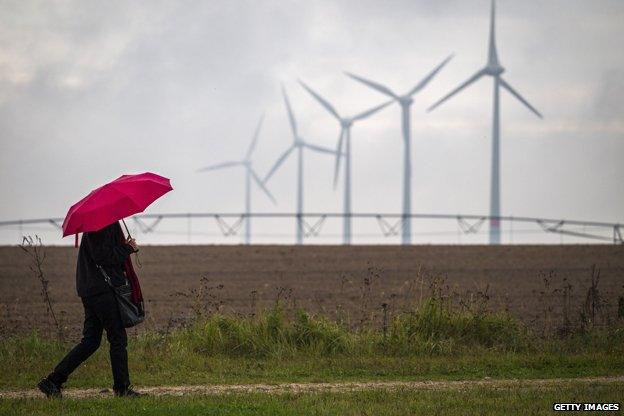
Wind and solar power, he says, are not enough: "If we had an all-renewable energy grid, sometimes we'd see the lights go out."
His argument is that even if we could build enough wind turbines and install enough solar panels to meet demand, we'd still need an alternative for when the sun isn't shining or the wind isn't blowing. We don't yet have the technology to store large amounts of power, so typically that means firing up expensive and polluting gas-fired power stations.
Most countries balance these variable sources of power with plants designed to operate almost continuously. The idea is that these steady, dependable power stations provide what is known as the "baseload", the minimum level of energy demand.
According to Mark Lynas, gas is too expensive for many countries so there are two main choices for baseload power: coal or nuclear.
Sizewell produces 1,200 MW of electricity.

More from Elementary Business
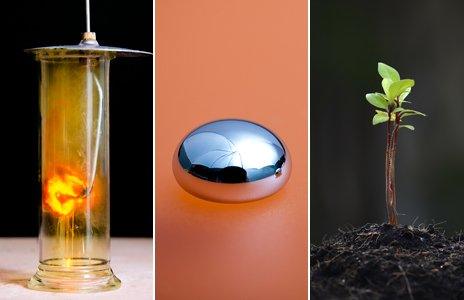

To generate the same amount of power, a coal-fired power station would have had to have burned something like 40m tonnes of coal. That would, in turn, have meant the emission of around 150m tonnes of CO2. The CO2 emissions are so much higher because coal is up to 80% carbon and, when CO2 is created, each carbon atom combines with two heavier oxygen atoms.
It is the very low carbon emissions from nuclear power that explain why Mark Lynas declares it to be a "green technology".
Hans-Josef Fell, the energy spokesman for the German greens, gasps in anger.
"Oh my God," he sputters, clearly astonished by the claim. "Look to the people who have lost their homelands."
Fell argues that the story of nuclear power is the story of a series of catastrophic accidents: the partial meltdown of a reactor at Three Mile Island in Pennsylvania in 1979, the reactor at Chernobyl in Ukraine that blew up in 1986 spreading radioactive material across northern Europe and, more recently, the disaster at Fukushima.
The UK's Sellafield nuclear reprocessing site became notorious in the first postwar decades for releasing radioactive waste into the Irish Sea.
Opponents of nuclear power like Hans-Josef Fell argue that these accidents have caused many tens of thousands of deaths, and many more cases of cancer and other illnesses.
The nuclear power industry says the number of deaths is wildly exaggerated. Lynas, for instance, argues there is no evidence of anyone dying from radiation at Fukushima and that more people die each day from coal pollution than have been killed by nuclear power during its 50 years of operation.
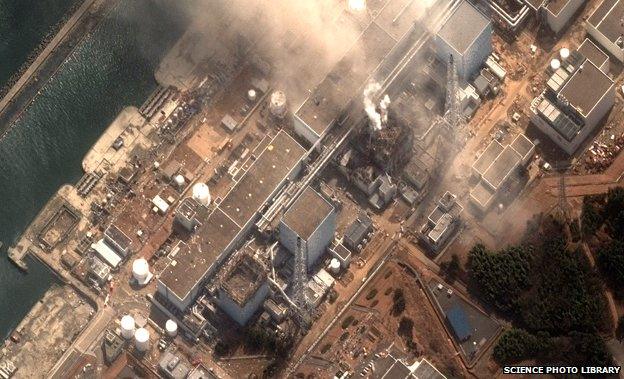
The average coal-fired power station, he claims, produces more radiation than the average nuclear power plant because of the traces of uranium and thorium in coal - not to mention other toxic ingredients such as mercury and lead.
Nevertheless, radioactivity remains for many an unusually terrifying concept.
And, that's why the campaign against nuclear power has been very successful in many countries. In the wake of Fukushima the German government decided to close down all its nuclear power stations by 2022 - despite the minuscule earthquake risk in central Europe.
Fell supports the decision. He accepts that climate change is a serious problem but argues that you "can't solve one problem with another problem".
Mark Lynas, on the other hand, describes the decision as a "disaster", and the reason why carbon emissions have actually risen in Germany, despite its huge investment in solar and wind.
But radiation isn't the only danger posed by nuclear power. There's another whole category of risk that needs to be taken into account: economic risk.
Building nuclear power stations is a very expensive business.
The "third generation" of nuclear reactors are supposed to be safe, affordable and suitable for mass production. Yet the first such plant, being built at Olkiluoto in Finland by the French company Areva, is many years behind schedule and many billions of euros over budget.
And nuclear power stations aren't just expensive to build, they are expensive to close down too.
A few hundred metres away from Sizewell B is the Sizewell A plant. The reactor was switched off in 2006 yet Tim Watkins, who is overseeing the decommissioning of the plant, doesn't think his work will be done until 2027.
Storing the waste is also pricey: the spent fuel in the fuel pond at Sizewell B will remain dangerously radioactive for tens of thousands of years.
All these costs explain why no nuclear power station has ever been built without some form of government subsidy.
And that leads to one last risk that must be considered when considering whether uranium is a suitable source of power.
Nuclear power stations last a very long time - at least 40 years. The question is whether it is wise to lock yourself into a relatively expensive and potentially dangerous form of power generation for such a long time.
Within 40 years the current gigantic research effort into batteries and other large-scale energy storage technologies may make renewables a viable alternative for baseload power, making nuclear power redundant.
If that happens the world may then stop trying to juggle the complex calculus of hazards and benefits created by the remarkable properties of this energetic atom.
The danger posed by nuclear weapons, however, will never go away.
Subscribe to the BBC News Magazine's email newsletter to get articles sent to your inbox.